Glial cells, also called glial cells or neuroglia, are cell which are non-neuronal and are located within the central nervous system and the peripheral nervous system that provides physical and metabolic support to neurons, including neuronal insulation and communication, and nutrient and waste transport.
Glial cells are a general term for many types of glial cells, for example, microglial, astrocytes, and Schwann cells, each having their own functions within the body. Each type of glial cell performs specific jobs that keep the brain functioning.
Primarily, glial cells provide support and protection to the neurons (nerve cells), maintain homeostasis, clean up debris, and form myelin. They essentially work to care for the neurons and the environment they are in.
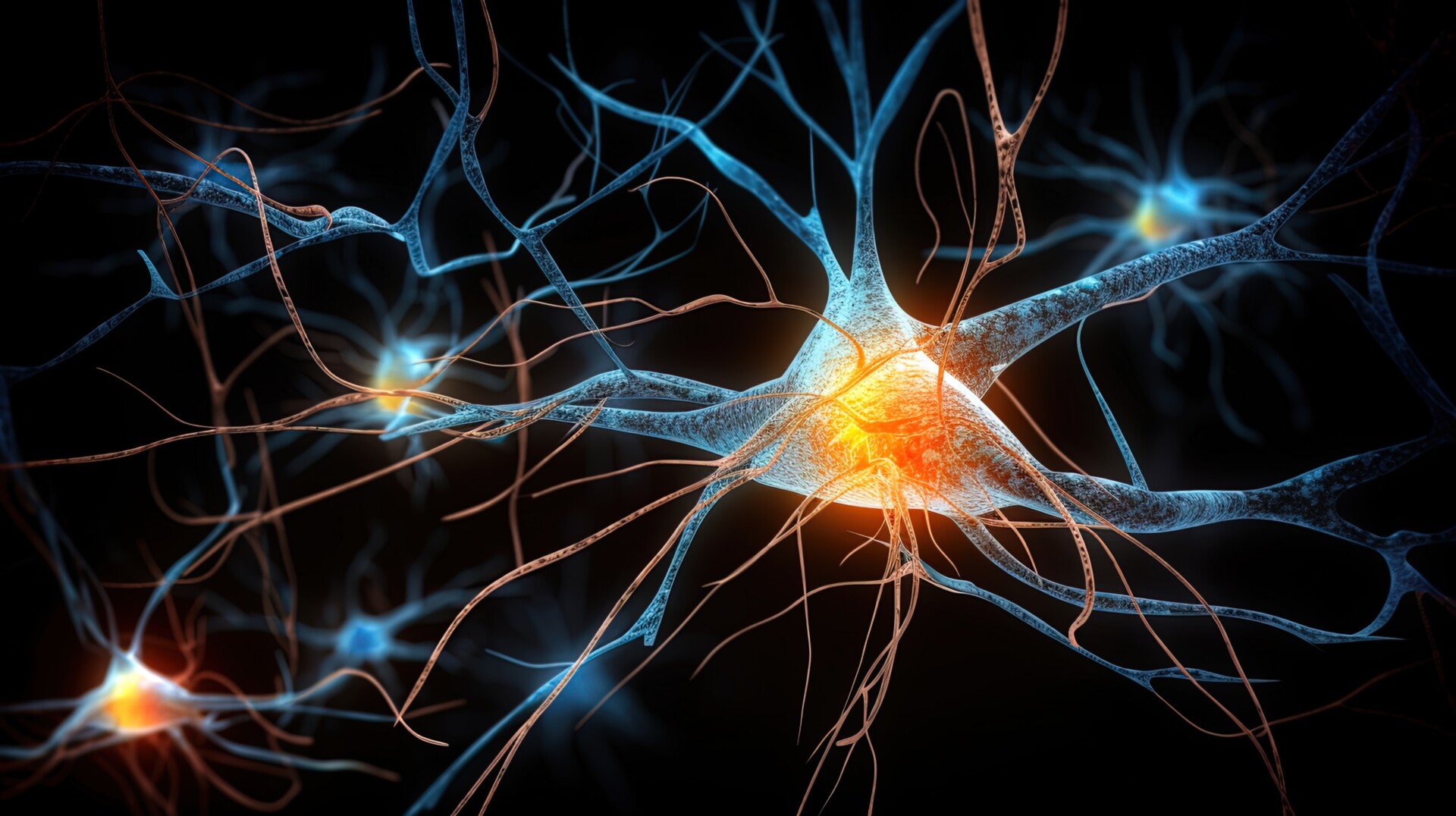
It is believed that German biologist Rudolf Virchow was the first to discover glial cells in 1856. Whilst looking for connective tissue in the brain, Virchow identified substances connected to the neurons.
This material was given the name nervenkitt in German, and neuroglial in Greek, which both translate to nerve glue. It was understood that glial cells only functioned as glue for the neurons, having a passive role to the neurons’ active role in the brain.
Some later researchers proposed that glial cells fed the neurons, whilst others believed they might be insulators for the electrical activity of the neurons.
Now, we have a more thorough understanding of the glia’s role in the brain, that they serve active and important functions in the overall maintenance of the brain and peripheral areas.
Glial cells differ from neurons in terms of structure. Neurons will have an axon and dendrites to transfer electrical signals between other nerve cells. Glial cells, however, do not have axons or dendrites.
This means that glial cells do not participate directly in synaptic interactions and electrical signaling, although they are supportive in helping the neurons maintain these functions.
Also, although glial cells have complex extensions from their cell bodies since they do not have axons or dendrites, this makes them typically smaller than neurons. Astrocytes, which are the largest type of glial cell, have a diameter of 40-50 microns.
Types of glial in the central nervous system (CNS)
Astrocytes
Astrocytes are star-shaped cells found only in the brain and spinal cord. Their main role is to maintain the environment around nerve cells to support signaling between them.
They do this by regulating neurotransmitter levels around synapses (connections between nerve cells). Astrocytes can sense neurotransmitter levels and release molecules that directly influence nerve cell activity. This makes them important for modifying synapses and how nerve cells communicate.
For example, glutamate is an excitatory neurotransmitter that stimulates brain activity. Astrocytes recycle excess glutamate to prevent overstimulation of neurons.
Astrocytes also recycle leftover neurotransmitters after a nerve signal is transmitted.
They additionally clean up debris when a nerve cell dies and remove excess potassium ions. Potassium is a chemical that is important for nerve function.
Furthermore, astrocytes help form the blood-brain barrier. This barrier filters out harmful substances to keep the brain healthy. Astrocytes also store blood sugar to provide fuel for nerve cells and help regulate metabolism in the brain.
When astrocytes are dysfunctional, they can no longer nurture neurons properly.
Astrocyte dysfunction has been linked to neurodegenerative diseases like ALS, Huntington’s disease, and Parkinson’s disease (Phatnani & Maniatis, 2015).
Astrocytes also become “reactive” in these diseases, changing their shape and behavior. This can be both good and bad. It may help isolate damaged areas but also release harmful inflammatory chemicals that damage neurons (Liddelow et al., 2017).
Oligodendrocytes
Oligodendrocytes form myelin sheaths around nerve cell axons in the brain and spinal cord. Myelin sheaths are made of fats and provide insulation for axons, which are long thread-like parts of nerve cells.
This insulation allows electrical signals to travel faster along axons.
Without myelin, signals would be much slower, leading to impaired brain function. Diseases like multiple sclerosis damage myelin. Oligodendrocytes also provide nutrients from the blood to axons.
Other diseases linked to problems with oligodendrocytes include leukodystrophies, which affect myelin, and the brain tumor oligodendroglioma.
Abnormal myelin formation has also been associated with mental health conditions such as schizophrenia (Scheel et al., 2013), with reduced oligodendrocyte density found in the prefrontal cortex and hippocampus of those with this disorder (Schmitt et al., 2019).
Oligodendrocyte dysfunction has also been associated with bipolar disorder (Konradi et al., 2012).
Microglial
Microglia act as the immune cells for the brain and spinal cord. They monitor for injury and disease and respond by clearing out dead cells, and removing toxins or invading organisms.
This inflammatory response helps heal injuries but can sometimes cause problems. For example, in Alzheimer’s disease microglia may become overactive, resulting in too much inflammation.
Microglia also play roles in brain development and plasticity. They prune unnecessary connections between nerve cells, leaving only the most important ones.
Dysfunction has been linked to conditions like chronic pain and fibromyalgia (Ohgidani et al., 2017).
An increased density and activation of microglia have been found in the brains of people with Autism spectrum disorders (ASD) compared to neurotypical brains, suggesting neuroinflammation may play a role in ASD (Tetreault et al., 2012).
Ependymal cells
Ependymal cells line the passageways in the brain called ventricles, as well as the central canal of the spinal cord. These cells form a thin membrane called the ependyma.
In the ventricles, ependymal cells have tiny hair-like projections called cilia that move back and forth to circulate cerebrospinal fluid.
Cerebrospinal fluid (CSF) carries nutrients to the brain and spinal cord while removing waste. It also acts as a cushion to protect the brain and maintain homeostasis by regulating temperature. Ependymal cells produce this important fluid.
If ependymal cells are damaged, it can disrupt the production and flow of cerebrospinal fluid. This allows waste to build up and makes it harder for nutrients to reach the brain and spinal cord. It also removes the cushioning effect, increasing the risk of injury.
Ependymal dysfunction has been associated with hydrocephalus, a condition involving excessive fluid buildup in the brain (Ji et al., 2022), as well as multiple sclerosis (Hatrock et al., 2020).
Compromised ependymal cell function can lead to altered CSF composition and impaired waste clearance. In diseases like Alzheimer’s, impaired CSF flow suggests ependymal cells fail to properly clear waste. This may promote further neurodegeneration (Nelles & Hazrati, 2022).
Radial glial
Radial glia are considered a type of stem cell in the brain, meaning they can generate new cells. They serve as the precursors that create neurons, astrocytes, and oligodendrocytes.
When you were an embryo, radial glia provided scaffolding to guide new brain cells into place during development.
Radial glia also contributes to the brain’s plasticity and ability to adapt later in life. Their role as stem cells has made them an area of interest for researchers looking into repairing brain damage from injury or illness.
Since radial glia creates other important brain cells, damage to them can impair neuron production and function. This could potentially contribute to neurodegenerative disorders.
Their dysfunction may also reduce the brain’s ability to form new connections, impacting learning.
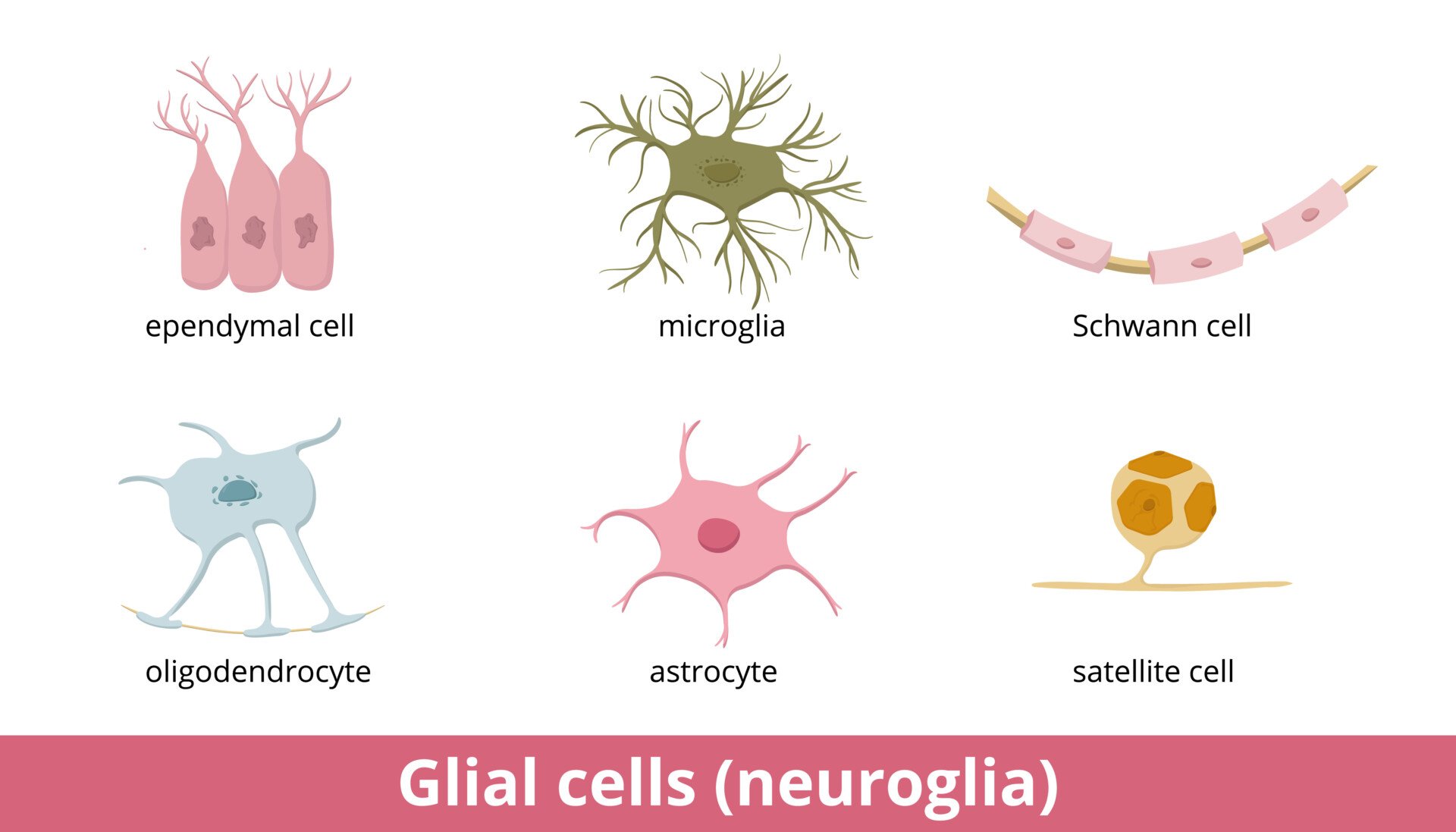
Types of glial in the peripheral nervous system (PNS)
Schwann cells
Schwann cells provide myelin insulation for axons of nerve cells in the peripheral nervous system, similar to oligodendrocytes in the central nervous system.
The membrane of Schwann cells spirals around axons to form the myelin sheath. This allows faster transmission of electrical signals along the nerves.
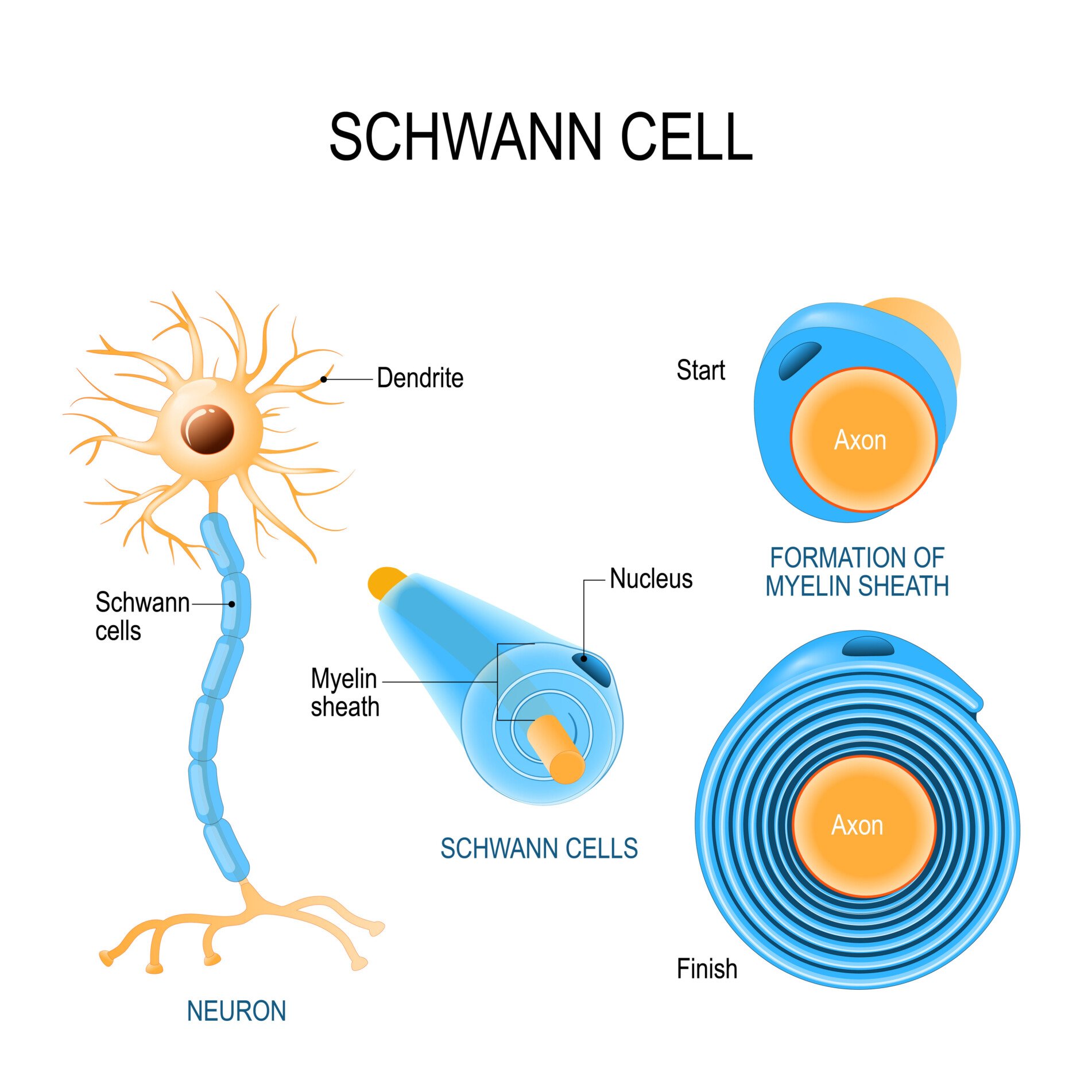
Unlike oligodendrocytes, each Schwann cell myelinates only one axon. Schwann cells are also involved in regenerating damaged peripheral nerves. When injury occurs, they help clear debris and provide a path for new axon fibers to regrow.
Abnormal myelination by Schwann cells can lead to loss of function in the peripheral nervous system. This may cause numbness, weakness, paralysis, or pain signals.
Diseases involving Schwann cells include Guillain-Barre syndrome, which causes peripheral nerve damage, and Charcot-Marie-Tooth disease, which is an inherited peripheral neuropathy.
Research suggests dysfunction in Schwann cells causes stress in their internal components, which could explain how they trigger harmful inflammation in some neuropathies. This presents a possible target for new treatments (Ydens et al., 2013).
Satellite cells
Satellite cells are located in sensory, sympathetic, and parasympathetic ganglia, which are clusters of nerve cell bodies.
They surround the nerve cell bodies in these ganglia. Satellite cells are thought to be similar to astrocytes in the central nervous system.
Their main role seems to be regulating the environment around nerve cells, including nutrient support and protection.
They may absorb toxins to prevent damage to the neurons. Satellite cells also respond to injury and inflammation like microglia do.
Dysfunction in satellite cells could disrupt the ganglia they are located in. For example, in sensory ganglia this could potentially affect senses like vision, hearing or touch.
Their dysfunction may also impair communication between the brain and internal organs controlled by the autonomic nervous system.
Mutations in genes involved in satellite cell function may directly cause muscle disorders known as “primary satellite cell-opathies,” where muscle weakness and wasting arise primarily from dysfunction of the muscle stem cells (Servian-Morilla et al., 2021).
Even in muscle disorders not directly caused by satellite cell mutations, increasing muscle damage can still indirectly impair satellite cell regeneration, as likely occurs in motor neuron diseases like ALS and spinal muscular atrophy (Ganassi et al., 2022).
FAQs
What do glial cells do?
Glial cells are non-neuronal cells that provide support and protection for neurons in the central nervous system.
They regulate neurotransmitters, isolate neurons, destroy pathogens, guide neuron migration during development, promote synaptic plasticity, and remove dead neurons.
Glial cells are crucial for the proper functioning of the nervous system.
Do glial cells produce myelin?
Yes, certain types of glial cells called oligodendrocytes and Schwann cells produce the myelin sheath around neuronal axons in the central and peripheral nervous systems, respectively.
Myelin acts as an insulating layer that increases the speed of neural signaling by preventing leakage of electrical impulses out of the axon.
Myelin production by glial cells is crucial for proper neuronal function and communication.
Why are glial cells important for neurons and brain function?
Glial cells are crucial because they help maintain the microenvironment neurons require to function properly.
They provide nutrients and energy to neurons, regulate neurotransmitter levels, insulate axons, and protect neurons from damage and infection.
Can glial cell dysfunction impact mental health?
Yes, emerging research implicates glial cell abnormalities in conditions like depression, anxiety, schizophrenia, and bipolar disorder.
Dysfunctional astrocytes and microglia likely contribute to inflammation that damages neurons.
Could targeting glial cells lead to new treatments for neurodegenerative diseases?
Potentially. Research on manipulating reactive astrocytes and microglia to reduce inflammation in diseases like Alzheimer’s looks promising.
Enhancing oligodendrocytes may also help repair myelin damage. More studies are needed.
References
Ganassi, M., & Zammit, P. S. (2022). Involvement of muscle satellite cell dysfunction in neuromuscular disorders: Expanding the portfolio of satellite cell-opathies. European Journal of Translational Myology, 32(1).
Hatrock, D., Caporicci-Dinucci, N., & Stratton, J. A. (2020). Ependymal cells and multiple sclerosis: proposing a relationship. Neural regeneration research, 15(2), 263.
Jäkel, S., & Dimou, L. (2017). Glial cells and their function in the adult brain: a journey through the history of their ablation. Frontiers in cellular neuroscience, 11, 24.
Ji, W., Tang, Z., Chen, Y., Wang, C., Tan, C., Liao, J., … & Xiao, G. (2022). Ependymal cilia: Physiology and role in hydrocephalus. Frontiers in Molecular Neuroscience, 15, 927479.
Konradi, C., Sillivan, S. E., & Clay, H. B. (2012). Mitochondria, oligodendrocytes and inflammation in bipolar disorder: evidence from transcriptome studies points to intriguing parallels with multiple sclerosis. Neurobiology of disease, 45(1), 37-47.
Liddelow, S. A., Guttenplan, K. A., Clarke, L. E., Bennett, F. C., Bohlen, C. J., Schirmer, L., … & Barres, B. A. (2017). Neurotoxic reactive astrocytes are induced by activated microglia. Nature, 541(7638), 481-487.
Nelles, D. G., & Hazrati, L. N. (2023). The pathological potential of ependymal cells in mild traumatic brain injury. Frontiers in Cellular Neuroscience, 17, 1216420.
Ohgidani, M., Kato, T. A., Hosoi, M., Tsuda, M., Hayakawa, K., Hayaki, C., … & Kanba, S. (2017). Fibromyalgia and microglial TNF-α: translational research using human blood induced microglia-like cells. Scientific reports, 7(1), 11882.
Phatnani, H., & Maniatis, T. (2015). Astrocytes in neurodegenerative disease. Cold Spring Harbor perspectives in biology, 7(6), a020628.
Purves, D. A. GJ., Fitzpatrick, D., et al. (2001). Neuroscience 2nd edition. Neuroglial cells.
Scheel, M., Prokscha, T., Bayerl, M., Gallinat, J., & Montag, C. (2013). Myelination deficits in schizophrenia: evidence from diffusion tensor imaging. Brain Structure and Function, 218, 151-156.
Servián-Morilla, E., Cabrera-Serrano, M., Johnson, K., Pandey, A., Ito, A., Rivas, E., … & Paradas, C. (2020). POGLUT1 biallelic mutations cause myopathy with reduced satellite cells, α-dystroglycan hypoglycosylation and a distinctive radiological pattern. Acta neuropathologica, 139, 565-582.
Schmitt, A., Simons, M., Cantuti-Castelvetri, L., & Falkai, P. (2019). A new role for oligodendrocytes and myelination in schizophrenia and affective disorders?. European Archives of Psychiatry and Clinical Neuroscience, 269, 371-372.
Tetreault, N. A., Hakeem, A. Y., Jiang, S., Williams, B. A., Allman, E., Wold, B. J., & Allman, J. M. (2012). Microglia in the cerebral cortex in autism. Journal of Autism and Developmental Disorders, 42(12), 2569-2584.
Ydens, E., Lornet, G., Smits, V., Goethals, S., Timmerman, V., & Janssens, S. (2013). The neuroinflammatory role of Schwann cells in disease. Neurobiology of disease, 55, 95-103.