On This Page:
In psychology, a synapse is the junction between two neurons where information is transmitted from one neuron to another. It consists of the axon terminal of the sending neuron, the synaptic cleft (a small gap), and the dendrite or cell body of the receiving neuron. Neurotransmitters are released across this gap to convey signals between the neurons.
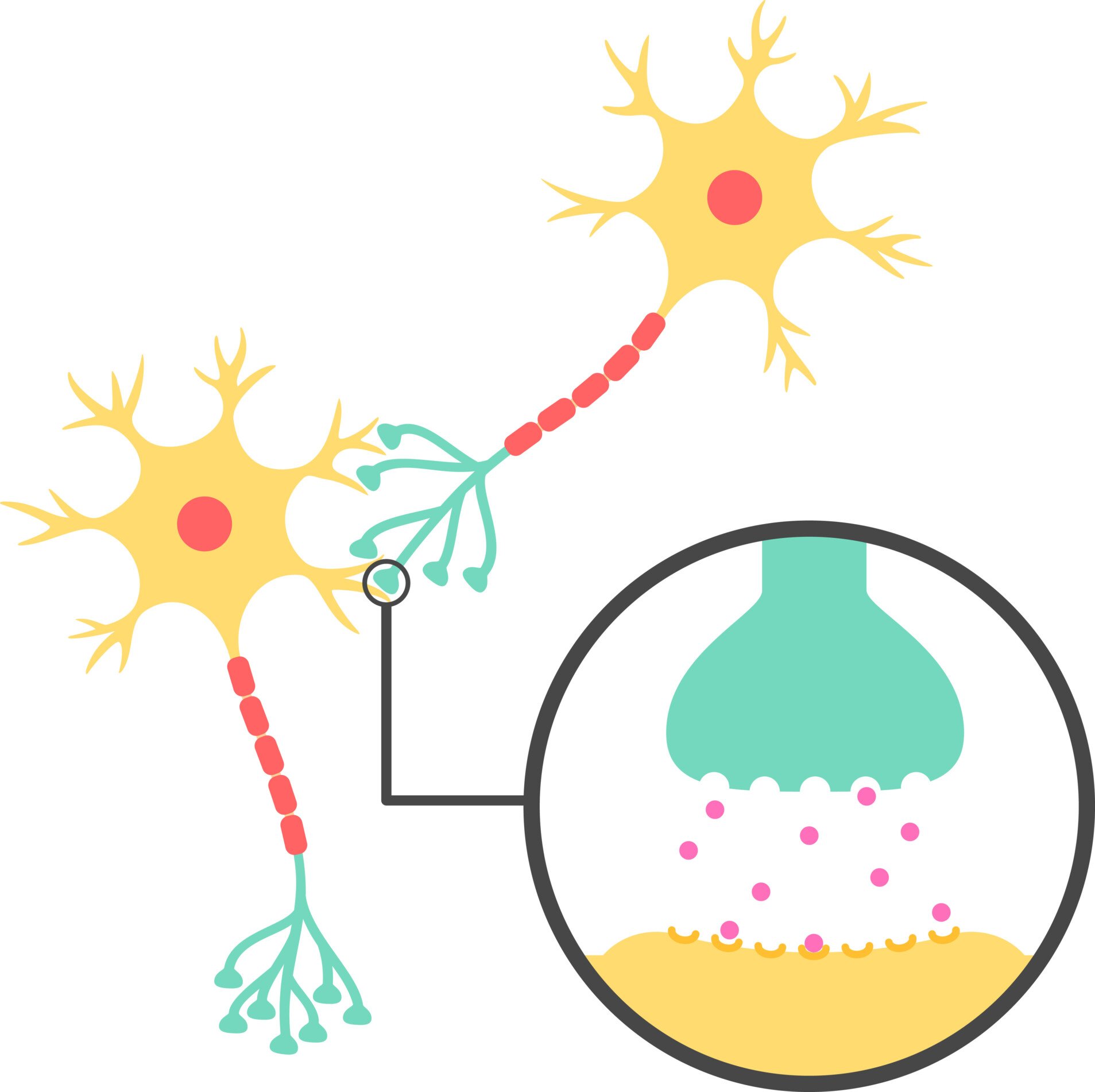
Key Points
- A synapse is a small gap between two neurons, where nerve impulses are relayed by a neurotransmitter from the axon of a presynaptic (sending) neuron to the dendrite of a postsynaptic (receiving) neuron. It is referred to as the synaptic cleft or synaptic gap.
- During synaptic transmission, the action potential (an electrical impulse) triggers the synaptic vesicles of the pre-synaptic neuron to release neurotransmitters (a chemical message).
- These neurotransmitters diffuse across the synaptic cleft (the gap between the pre and post-synaptic neurons) and bind to specialized receptor sites on the post-synaptic neuron.
- If the neurotransmitter is excitatory (e.g., noradrenaline), then the post-synaptic neuron is more likely to fire an impulse. If the neurotransmitter is inhibitory (e.g., serotonin ), then the post-synaptic neuron is less likely to fire an impulse.
- The excitatory and inhibitory influences are summed to determine whether/how frequently the neuron will fire (summation). At the dendrites, the chemical message is converted back into an electrical impulse and the process of transmission occurs again.
What is a Synapse?
Neurons do not touch each other, but where the neuron does come close to another neuron, a synapse is formed between the two. This is how neurons communicate with each other.
Neurons essentially communicate with each other through synapses. When signals have traveled through neurons to the endpoint, they cannot simply continue onto the next neuron.
It must trigger the release of neurotransmitters which then carry the signals across the synapse to reach the next neuron. Terminal buttons belong to the presynaptic endings of the neuron and have vessels containing neurotransmitters. These are responsible for transmitting signals to other neurons.
When a nerve impulse has triggered the release of these neurotransmitters from the terminal buttons, these chemicals are then released into the synaptic cleft and are then taken up by receptors on the next cell.
The neuron that then receives the neurotransmitters is the postsynaptic neuron. Neurons receive messages from many terminal buttons, and in turn, terminal buttons form synapses with many other neurons.
A synapse is a combination of the following:
- Presynaptic endings – which contain the neurotransmitters (chemical messengers).
- Synaptic clefts – which is the gap between the two neurons, typically about 20 to 30 nanometers wide.
- Postsynaptic endings – which contain the sites for receptors (molecules that receive signals for a cell).
Synapses also have the ability to communicate a change in the message being passed on. Postsynaptic neurons can also send communications back to the presynaptic neurons telling them to change how often or much a neurotransmitter is released.
Due to this, we can say that the synapses can communicate bi-directionally.
Synaptic Transmission
Synapses can be either chemical or electrical and are essential to the functioning of neural activity. Neuroscientists understand that synapses play a vital role in a variety of cognitive functions, including learning and memory formation.
Chemical Synapses | Electrical Synapses |
---|---|
Gap between cells is about 20 nanometres | Gap between cells is about 3.5 nanometres |
Speed of transmission is several milliseconds | Speed of transmission is nearly instantaneous |
Can be excitatory or inhibitory | Excitatory only |
No loss of signal strength | Signal strength diminishes over time |
Chemical Synaptic Transmission
The most commonly found synapses in humans are chemical synapses. This occurs due to electrical activity in the presynaptic neurons triggering the release of neurotransmitters.
The neurotransmitters disperse across the synaptic cleft to then bind themselves to specialized receptors of postsynaptic neurons. Once this occurs, the neurotransmitters then either excite or inhibit the postsynaptic neuron.
Exciting the postsynaptic neuron leads to a firing of action potential (electrical impulses), whereas inhibiting the postsynaptic neuron prevents the transmission of a signal.
Inside the presynaptic neuron are synaptic vesicles, which are covered in a membrane and contain neurotransmitters.
When an action potential arrives at the presynaptic terminal, it activates voltage-gated calcium channels (Ca² +) in the neuron’s membrane. Ca² + are highly concentrated on the outside of the neuron and will rush into the neuron when activated.
The Ca² + permits the synaptic vesicles to fuse with the presynaptic terminal’s membrane, enabling it to release neurotransmitters into the synaptic cleft.
The transmitter molecules then will diffuse across the synaptic cleft and will bind to the receptors of the postsynaptic neuron. When these receptors are activated, this leads to either the opening or closing of ion channels, which are membrane proteins that provide a passageway through which charged ions can cross.
Depending on the ions involved, this may either be depolarising- making the inside of the cell more positive, or hyperpolarisation – which makes the inside of the cell more negative (less likely for an action potential to be generated in the postsynaptic neuron).
Electrical Synaptic Transmission
Electrical synapses are different from chemical synapses as there is a direct physical connection between the presynaptic and the postsynaptic neuron. This connection takes the form of something called a gap junction, which is essentially a channel that allows ions to flow directly from the presynaptic cell to the postsynaptic cell.
Gap junctions contain paired channels in the membranes of the pre and postsynaptic neurons, forming pores. These pores are larger than those of the voltage-gated ion channels in chemical synapses, meaning that a variety of substances can diffuse between the neurons.
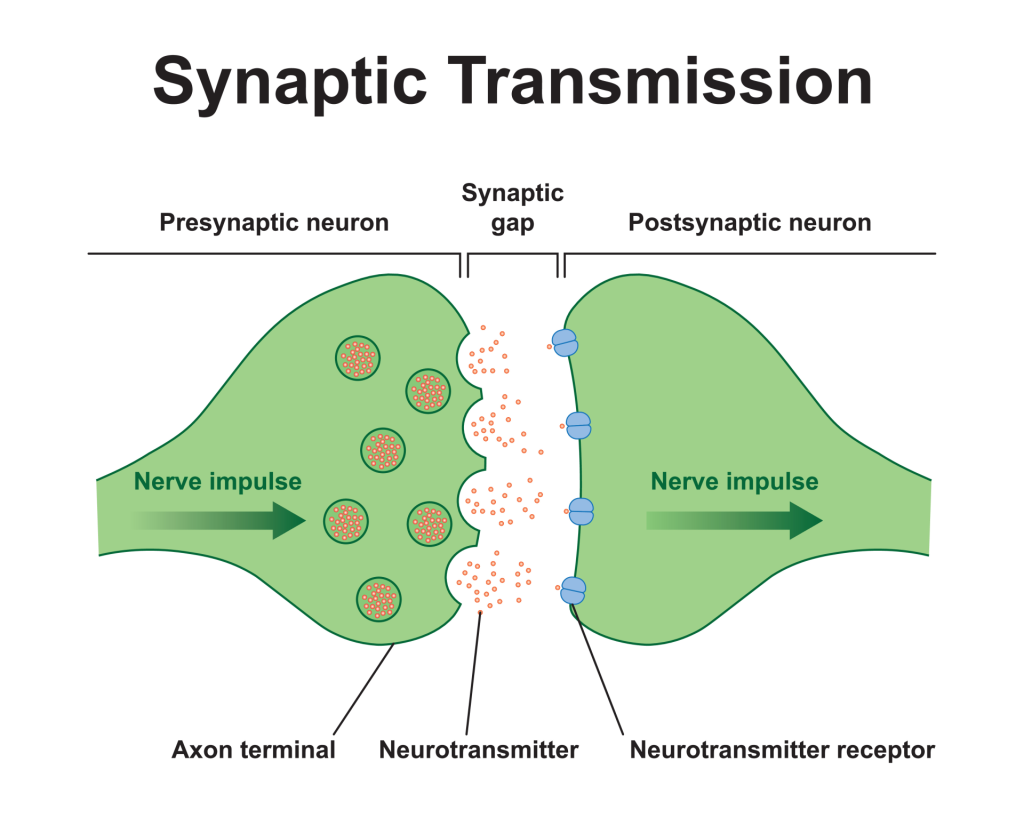
Electrical synapses transmit signals much quicker than chemical synapses, almost instantaneously, compared with chemical synapses taking several milliseconds.
Despite being a lot faster to transmit signals, electrical synapses’ signal strength is diminished over time, whereas chemical synapses do not lose their signal strength. Also, whilst chemical synapses can be excitatory or inhibitory, electrical synapses can only be excitatory.
Excitatory and Inhibitory Postsynaptic Potentials
- The effect of the presynaptic neuron on the postsynaptic neuron can be either excitatory or inhibitory.
- Neurotransmitters are chemical messengers that are released from a synaptic vesicle into the synapse by neurons.
- Inhibitory neurotransmitters decrease the likelihood of the neuron firing. They are generally responsible for calming the mind
and inducing sleep. This is the case for serotonin. - Excitatory neurotransmitters increase the likelihood that an excitatory signal is sent to the post-synaptic cell. Adrenalin is which is both a neurotransmitter and a hormone has an excitatory effect.
Chemicals released from the presynaptic neuron may either excite or inhibit the postsynaptic neuron, telling it to release neurotransmitters or to slow down or stop signaling.
When the axon fires and the terminal buttons release a neurotransmitter that excites the postsynaptic neuron, this is excitatory postsynaptic potential (EPSP). This effect of excitation makes it more likely that the axons of the postsynaptic neuron will also fire.
The inhibitory postsynaptic potential (IPSP) has the opposite effect. Inhibition is caused by inhibitory neurotransmitters. When the neurotransmitter binds with the post-synaptic receptor, it results in a IPSP, and the cell is less likely to fire.
The rate at which the axon fires is determined by the activity of the synapses on the dendrites and soma of the neuron. If the excitatory synapses are more active, then the axons fire at a high rate, compared to firing at a low rate, or not at all when inhibitory synapses are active.
An EPSP is depolarising, meaning it makes the inside of the neuron more positive, which causes more action potential. IPSPs, however, bring the potential down, meaning it will be less likely to cause action potential and can cancel out the excitatory effect of the EPSPs.
Spatial and Temporal Summation
Summation is the process that determines whether/how frequently the neuron will fire
by the combined effects of excitatory and inhibitory signals, both from multiple simultaneous inputs (spatial summation) and from repeated inputs (temporal summation).
EPSPs and IPSPs interact with each other when a postsynaptic neuron combines (or summates) all the excitatory and inhibitory signals it receives and then makes a decision as to whether to fire an action potential. There are two types of summation that can occur at this stage:
- Spatial summation – this happens when all the postsynaptic potentials occur in different locations but at about the same time.
- Temporal summation – this happens when all the postsynaptic potentials occur in the same place but at slightly different times.
For example, if there are two single excitatory signals (EPSPs) that arrive at the postsynaptic neuron from two different dendrites, they cannot reach the threshold for action potential on their own. However, they can sum up together to reach the threshold and cause an action potential of the postsynaptic neuron.
If an inhibitory signal (IPSP) also enters through another dendrite, this can counteract the two EPSPs and could prevent the neuron from firing action potential. This is an instance of spatial summation.
If the same two EPSPs arrive from the presynaptic neuron to the postsynaptic neuron but at slightly different times, they can still cause an action potential to fire. This is because postsynaptic potentials are not instantaneous, and they can survive in a neuron for a while before dissipating.
Therefore, if one EPSP arrives first and has not dissipated yet when the second EPSP arrives, it can then reach the threshold for action potential. This is an instance of temporal summation.
Reuptake of Neurotransmitters
For a synapse to function effectively, it must be shut off once the signal is sent. This signal termination allows the postsynaptic neuron to return to its resting potential state, ready for new signals.
When neurotransmitters get released into the synaptic cleft, not all of them are able to attach to the receptors of the next neuron.
The synaptic cleft must be cleared of all neurotransmitters at signal termination, which can be done by either being broken down by enzymes, diffused away, or re-uptake occurs.
Re-uptake is when neurotransmitters get reabsorbed back into the presynaptic neuron from which they came from. Transporter proteins from the presynaptic membrane remove the neurotransmitters from the synaptic cleft, carrying the neurotransmitter back into the presynaptic neuron.
The neurotransmitter then either gets re-packaged into the synaptic vesicles and stored until it is next needed again, or they are broken down by enzymes.
Serotonin is a type of neurotransmitter that is associated with a variety of psychological and bodily functions, such as mood, sexual desire, appetite, sleep, and memory.
If there are imbalances in the way serotonin is transmitted between neurons through too much reuptake of this neurotransmitter, then this has implications for contributing to mood disorders, specifically depression.
Selective serotonin reuptake inhibitors (SSRIs) are a type of medication, also known as antidepressants, which work in a way to increase the amount of serotonin being transmitted between cells. SSRIs essentially aid in blocking the reuptake of serotonin into the presynaptic cell, meaning there is more serotonin in the synaptic cleft.
If there is more serotonin in the synaptic cleft, it is more likely that serotonin will reach the receptors of the postsynaptic cell. As SSRIs allow more serotonin to pass along between neurons, they have been shown to alleviate mood disorders, making them a common therapy for depression.
Synaptic Plasticity
Plasticity refers to how much something can be changed or adapted through growth and reorganization. It was once believed that once synapses were formed, they remained the same forever, never changing.
However, it is now understood that activity, or lack of activity, can affect the strength of synapses or even change the number and structure of synapses in the brain. Therefore, the more the synapses are used, the stronger they can become and the more influence they can have over postsynaptic neurons.
Likewise, not fully using synapses can weaken them and can have a detrimental impact over the long-term.
Further Reading
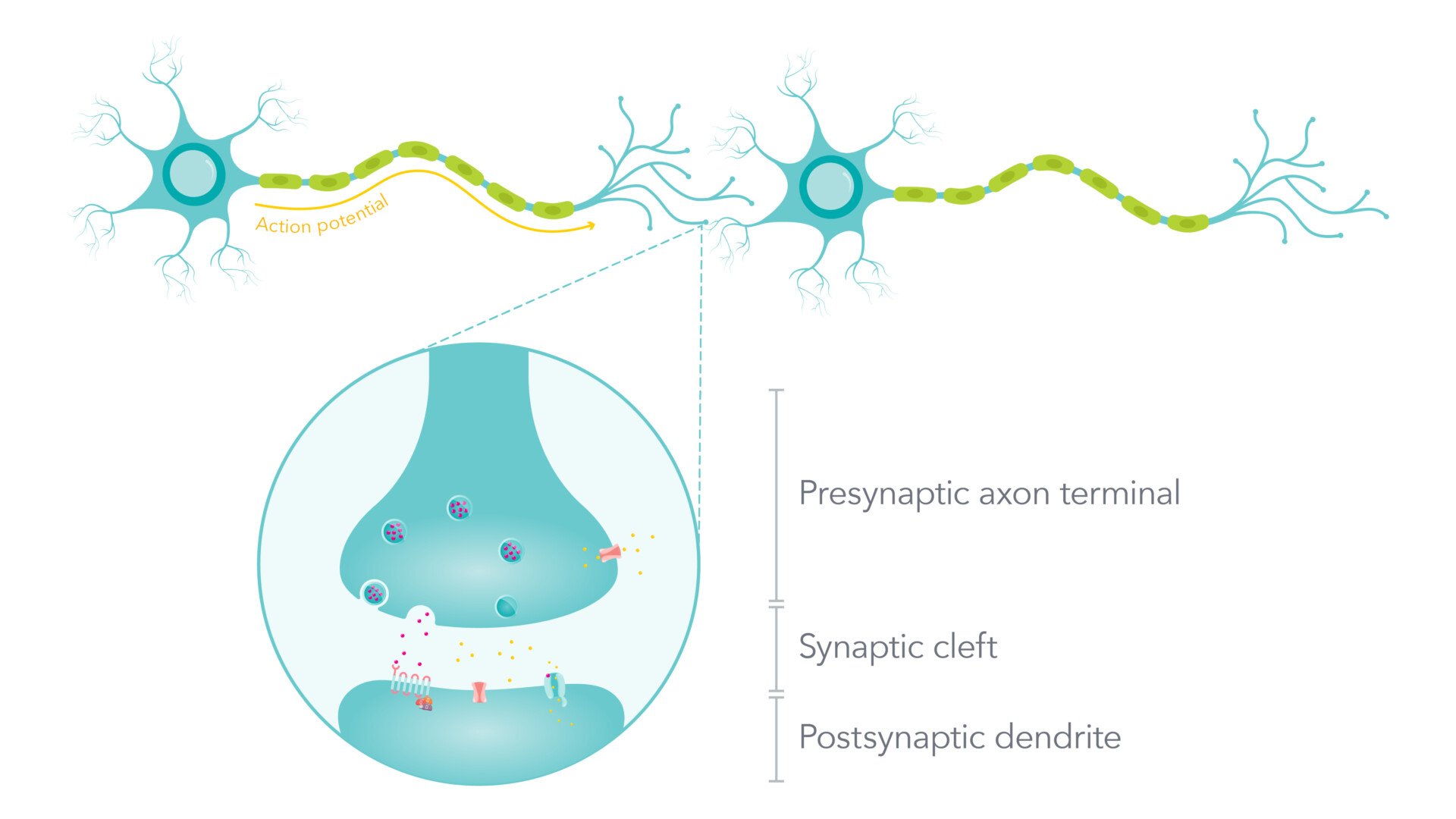
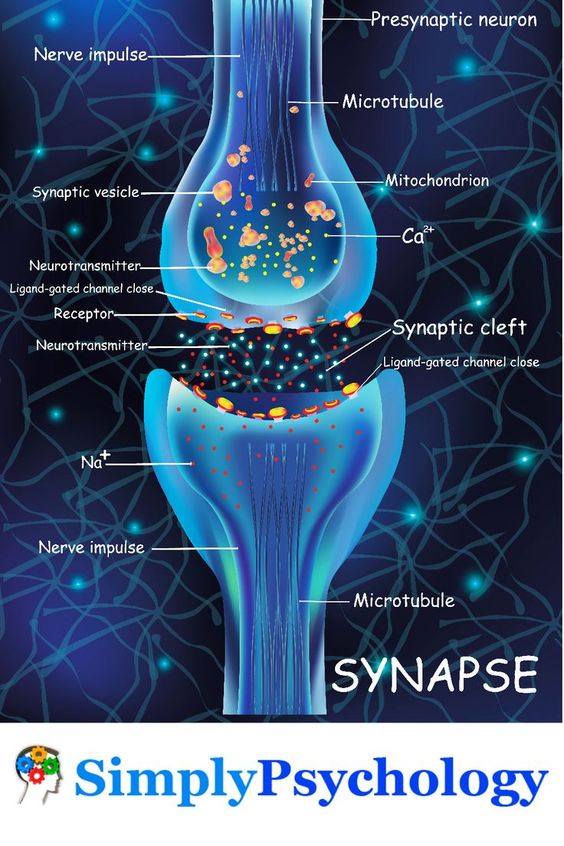